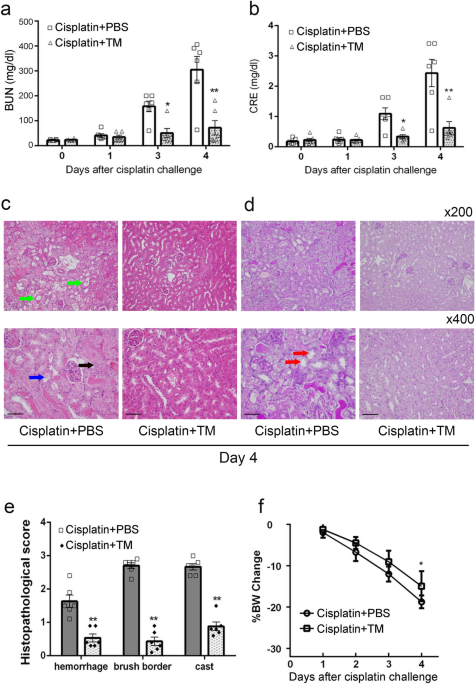
Hoste, E. A. J. et al. Global epidemiology and outcomes of acute kidney injury. Nat. Rev. Nephrol. 14(10), 607–625. https://doi.org/10.1038/s41581-018-0052-0 (2018).
Kellum, J. A., Ronco, C. & Bellomo, R. Conceptual advances and evolving terminology in acute kidney disease. Nat. Rev. Nephrol. 17(7), 493–502. https://doi.org/10.1038/s41581-021-00410-w (2021).
Chawla, L. S. et al. Acute kidney disease and renal recovery: Consensus report of the Acute Disease Quality Initiative (ADQI) 16 Workgroup. Nat. Rev. Nephrol. 13(4), 241–257. https://doi.org/10.1038/nrneph.2017.2 (2017).
Mehta, R. L. et al. Recognition and management of acute kidney injury in the International Society of Nephrology 0by25 Global Snapshot: A multinational cross-sectional study. Lancet 387(10032), 2017–2025. https://doi.org/10.1016/S0140-6736(16)30240-9 (2016).
Santos, N. A. G. D., Ferreira, R. S. & Santos, A. C. D. Ovreview of cisplatin-induced neurotoxicity and ototoxicity, and the protective agents. Food Chem. Toxicol. 136, 111079 (2020).
Ürün, M. et al. Evaluation of the healing and protective properties of adipose-derived mesenchymal stem cells from cisplatin-induced liver and kidney damage. Eur. Rev. Med. Pharmacol. Sci. 28(4), 1327–1339. https://doi.org/10.26355/eurrev_202402_35454 (2024).
Yamaguchi, T. et al. Recombinant soluble thrombomodulin attenuates cisplatin-induced intestinal injury by inhibiting intestinal epithelial cell-derived cytokine secretion. Mol. Biol. Rep. 50(10), 8459–8467. https://doi.org/10.1007/s11033-023-08762-1 (2023).
Volarevic, V. et al. Molecular mechanisms of cisplatin-induced nephrotoxicity: A balance on the knife edge between renoprotection and tumor toxicity. J. Biomed. Sci. 26(1), 25. https://doi.org/10.1186/s12929-019-0518-9 (2019).
Guo, Y. et al. MicroRNA-709 mediates acute tubular injury through effects on mitochondrial function. J. Am. Soc. Nephrol. 29(2), 449–461. https://doi.org/10.1681/ASN.2017040381 (2018).
Ichinomiya, M. et al. Demonstration of mitochondrial damage and mitophagy in cisplatin-mediated nephrotoxicity. Tohoku J. Exp. Med. 246(1), 1–8. https://doi.org/10.1620/tjem.246.1 (2018).
Jia, P. et al. Uncoupling protein 1 inhibits mitochondrial reactive oxygen species generation and alleviates acute kidney injury. EBioMedicine 49, 331–340. https://doi.org/10.1016/j.ebiom.2019.10.023 (2019).
Xu, C., Bailly-Maitre, B. & Reed, J. C. Endoplasmic reticulum stress: Cell life and death decisions. J. Clin. Investig. 115(10), 2656–2664. https://doi.org/10.1172/JCI26373 (2005).
Linkermann, A. et al. Regulated cell death in AKI. J. Am. Soc. Nephrol. 25(12), 2689–2701. https://doi.org/10.1681/ASN.2014030262 (2014).
Ishimoto, Y. & Inagi, R. Mitochondria: A therapeutic target in acute kidney injury. Nephrol. Dial. Transplant. 31(7), 1062–1069. https://doi.org/10.1093/ndt/gfv317 (2016).
Gallazzini, M. & Pallet, N. Endoplasmic reticulum stress and kidney dysfunction. Biol. Cell 110(9), 205–216. https://doi.org/10.1111/boc.201800019 (2018).
Hashimoto, S. et al. In vitro and in vivo release of cytostatic factors from Lactobacillus casei-elicited peritoneal macrophages after stimulation with tumor cells and immunostimulants. Cancer Immunol. Immunother. 24(1), 1–7. https://doi.org/10.1007/BF00199825 (1987).
Esmon, C. T. Thrombomodulin as a model of molecular mechanisms that modulate protease specificity and function at the vessel surface. FASEB. J. 9(10), 946–955. https://doi.org/10.1096/fasebj.9.10.19 (1995).
Wouwer, M., Collen, D. & Conway, E. M. Thrombomodulin-protein C-EPCR system: Integrated to regulate coagulation and inflammation. Arterioscler. Thromb. Vasc. Biol. 24(8), 1374–1383. https://doi.org/10.1161/01.ATV.0000134298.25489.92 (2004).
Cheng, T. L. et al. Thrombomodulin regulates keratinocyte differentiation and promotes wound healing. J. Investig. Dermatol. 133(6), 1638–1645. https://doi.org/10.1038/jid.2013.8 (2013).
Matsumoto, M. et al. Efficacy of recombinant human-soluble thrombomodulin for severe acute pancreatitis in a rat experimental model. Pancreas 49(4), 503–508. https://doi.org/10.1097/MPA.0000000000001527 (2020).
Kurokohchi, K., Imataki, O. & Kubo, F. Anti-inflammatory effect of recombinant thrombomodulin for fulminant hepatic failure. World J. Gastroenterol. 21(26), 8203–8207. https://doi.org/10.3748/wjg.v21.i26.8203 (2015).
Conway, E. M., Nowakowski, B. & Steiner-Mosonyi, M. Human neutrophils synthesize thrombomodulin that does not promote thrombin-dependent protein C activation. Blood 80(5), 1254–1263 (1992).
Conway, E. M. & Nowakowski, B. Biologically active thrombomodulin is synthesized by adherent synovial fluid cells and is elevated in synovial fluid of patients with rheumatoid arthritis. Blood 81(3), 726–733 (1993).
McCachren, S. S. et al. Thrombomodulin expression by human blood monocytes and by human synovial tissue lining macrophages. Blood 78(12), 3128–3132 (1991).
Ito, T. & Maruyama, I. Thrombomodulin: Protectorate God of the vasculature in thrombosis and inflammation. J. Thromb. Haemost. 9(Suppl 1), 168–173. https://doi.org/10.1111/j.1538-7836.2011.04319.x (2011).
Sharfuddin, A. A. et al. Soluble thrombomodulin protects ischemic kidneys. J. Am. Soc. Nephrol. 20(3), 524–534. https://doi.org/10.1681/ASN.2008060593 (2009).
Nakahara, M. et al. Recombinant thrombomodulin protects mice against histone-induced lethal thromboembolism. PLoS One 8(9), e75961. https://doi.org/10.1371/journal.pone.0075961 (2013).
Hazman, Ö. et al. The effect of boric acid and borax on oxidative stress, inflammation, ER stress and apoptosis in cisplatin toxication and nephrotoxicity developing as a result of toxication. Inflammation 41(3), 1032–1048. https://doi.org/10.1007/s10753-018-0756-0 (2018).
Havasi, A. & Borkan, S. C. Apoptosis and acute kidney injury. Kidney Int. 80(1), 29–40. https://doi.org/10.1038/ki.2011.120 (2011).
Manohar, S. & Leung, N. Cisplatin nephrotoxicity: A review of the literature. J. Nephrol. 31(1), 15–25. https://doi.org/10.1007/s40620-017-0392-z (2018).
Hao, Y. et al. 2-Methylquinazoline derivative 23BB as a highly selective histone deacetylase 6 inhibitor alleviated cisplatin-induced acute kidney injury. Biosci. Rep. 40(1), BSR20191538. https://doi.org/10.1042/BSR20191538 (2020).
Tan, Z. et al. Pharmacological and genetic inhibition of fatty acid-binding protein 4 alleviated cisplatin-induced acute kidney injury. J. Cell. Mol. Med. 23(9), 6260–6270. https://doi.org/10.1111/jcmm.14512 (2019).
Kim, H. J. et al. NLRP3 inflammasome knockout mice are protected against ischemic but not cisplatin-induced acute kidney injury. J. Pharmacol. Exp. Ther. 346(3), 465–472. https://doi.org/10.1124/jpet.113.205732 (2013).
Kimura, A. et al. Interferon-γ is protective in cisplatin-induced renal injury by enhancing autophagic flux. Kidney Int. 82(10), 1093–1104. https://doi.org/10.1038/ki.2012.240 (2012).
Burks, S. R. et al. Mesenchymal stromal cell potency to treat acute kidney injury increased by ultrasound-activated interferon-γ/interleukin-10 axis. J. Cell. Mol. Med. 22(12), 6015–6025. https://doi.org/10.1111/jcmm.13874 (2018).
Gozzelino, R., Jeney, V. & Soares, M. P. Mechanisms of cell protection by heme oxygenase-1. Annu. Rev. Pharmacol. Toxicol. 2010(50), 323–354. https://doi.org/10.1146/annurev.pharmtox.010909.105600 (2010).
Grochot-Przeczek, A., Dulak, J. & Jozkowicz, A. Haem oxygenase-1: Non-canonical roles in physiology and pathology. Clin. Sci. (Lond.) 122(3), 93–103. https://doi.org/10.1042/CS20110147 (2012).
Bolisetty, S. et al. Proximal tubule-targeted heme oxygenase-1 in cisplatin-induced acute kidney injury. Am. J. Physiol. Renal Physiol. 310(5), F385–F394. https://doi.org/10.1152/ajprenal.00335.2015 (2016).
Deng, J. S. et al. Cordyceps cicadae mycelia ameliorate cisplatin-induced acute kidney injury by suppressing the TLR4/NF-κB/MAPK and activating the HO-1/Nrf2 and Sirt-1/AMPK pathways in mice. Oxid. Med. Cell Longev. https://doi.org/10.1155/2020/7912763 (2020).
Chien, L. H. et al. Salvianolic acid C protects against cisplatin-induced acute kidney injury through attenuation of inflammation, oxidative stress and apoptotic effects and activation of the CaMKK-AMPK-Sirt1-associated signaling pathway in mouse models. Antioxidants (Basel) 10(10), 1620. https://doi.org/10.3390/antiox10101620 (2021).
Gao, L. et al. Restoration of E-cadherin by PPBICA protects against cisplatin-induced acute kidney injury by attenuating inflammation and programmed cell death. Lab. Investig. 98(7), 911–923. https://doi.org/10.1038/s41374-018-0052-5 (2018).
Ramesh, G. & Reeves, W. B. TNF-α mediates chemokine and cytokine expression and renal injury in cisplatin nephrotoxicity. J. Clin. Investig. 110(6), 835–842. https://doi.org/10.1172/JCI15606 (2002).
Faubel, S. et al. Cisplatin-induced acute renal failure is associated with an increase in the cytokines interleukin (IL)-1β, IL-18, IL-6, and neutrophil infiltration in the kidney. J. Pharmacol. Exp. Ther. 322(1), 8–15. https://doi.org/10.1124/jpet.107.119792 (2007).
Ding, Y. et al. Regulation networks of non-coding RNA-associated ceRNAs in cisplatin-induced acute kidney injury. Cells 11(19), 2971. https://doi.org/10.3390/cells11192971 (2022).
Ma, Z. et al. Single-nucleus transcriptional profiling of chronic kidney disease after cisplatin nephrotoxicity. Am. J. Pathol. 192(4), 613–628. https://doi.org/10.1016/j.ajpath.2021.12.012 (2022).
Peng, L., Xinxiu, L., Weixing, L. & Xu, Z. Inhibition of CXCL1-CXCR2 axis ameliorates cisplatin-induced acute kidney injury by mediating inflammatory response. Biomed. Pharmacother. 122, 109693. https://doi.org/10.1016/j.biopha.2019.109693 (2020).
Pabla, N. & Dong, Z. Cisplatin nephrotoxicity: Mechanisms and renoprotective strategies. Kidney Int. 73(9), 994–1007. https://doi.org/10.1038/sj.ki.5002786 (2008).
Mahgoub, E. et al. Genipin attenuates cisplatin-induced nephrotoxicity by counteracting oxidative stress, inflammation, and apoptosis. Biomed. Pharmacother. 93, 1083–1097. https://doi.org/10.1016/j.biopha.2017.07.018 (2017).
Landau, S. I. et al. Regulated necrosis and failed repair in cisplatin-induced chronic kidney disease. Kidney Int. 95(4), 797–814. https://doi.org/10.1016/j.kint.2018.11.042 (2019).
Gómez-Sierra, T. et al. Role of food-derived antioxidants against cisplatin induced-nephrotoxicity. Food Chem. Toxicol. 120, 230–242. https://doi.org/10.1016/j.fct.2018.07.018 (2018).
Chirino, Y. I. & Pedraza-Chaverri, J. Role of oxidative and nitrosative stress in cisplatin-induced nephrotoxicity. Exp. Toxicol. Pathol. 61(3), 223–242. https://doi.org/10.1016/j.etp.2008.09.003 (2009).
Cao, S. S. & Kaufman, R. J. Targeting endoplasmic reticulum stress in metabolic disease. Expert. Opin. Ther. Targets 17(4), 437–448. https://doi.org/10.1517/14728222.2013.756471 (2013).
Small, D. M., Coombes, J. S., Bennett, N., Johnson, D. W. & Gobe, G. C. Oxidative stress, anti-oxidant therapies and chronic kidney disease. Nephrology (Carlton) 17(4), 311–321. https://doi.org/10.1111/j.1440-1797.2012.01572.x (2012).
Almanza, A. et al. Endoplasmic reticulum stress signaling—From basic mechanisms to clinical applications. FEBS J. 286(2), 241–278. https://doi.org/10.1111/febs.14608 (2019).
Reyes-Fermín, L. M. et al. Natural antioxidants’ effects on endoplasmic reticulum stress-related diseases. Food Chem. Toxicol. 138, 111229. https://doi.org/10.1016/j.fct.2020.111229 (2020).
Goligorsky, M. S. Oxidative stress and the kidney: Riding on the curve of hormesis. Antioxid. Redox Signal. 25(3), 117–118. https://doi.org/10.1089/ars.2016.6794 (2016).
Lieberthal, W., Triaca, V. & Levine, J. Mechanisms of death induced by cisplatin in proximal tubular epithelial cells: Apoptosis vs necrosis. Am. J. Physiol. 270(42), F700–F708. https://doi.org/10.1152/ajprenal.1996.270.4.F700 (1996).
Breckenridge, D. G., Germain, M., Mathai, J. P., Nguyen, M. & Shore, G. C. Regulation of apoptosis by endoplasmic reticulum pathways. Oncogene 22(53), 8608–8618. https://doi.org/10.1038/sj.onc.1207108 (2003).
Xiao, B. et al. TTF1-NPs induce ERS-mediated apoptosis and inhibit human hepatoma cell growth in vitro and in vivo. Oncol. Res. 23(6), 311–320. https://doi.org/10.3727/096504016X14567549091341 (2016).
Marrer, E. & Dieterle, F. Impact of biomarker development on drug safety assessment. Toxicol. Appl. Pharmacol. 243(2), 167–179. https://doi.org/10.1016/j.taap.2009.12.015 (2010).
Liu, H. & Baliga, R. Endoplasmic reticulum stress-associated caspase 12 mediates cisplatin-induced LLC-PK1 cell apoptosis. J. Am. Soc. Nephrol. 16(7), 1985–1992. https://doi.org/10.1681/ASN.2004090768 (2005).
Márton, M. et al. A systems biological view of life-and-death decision with respect to endoplasmic reticulum stress—The role of PERK pathway. Int. J. Mol. Sci. 18(1), 58. https://doi.org/10.3390/ijms18010058 (2017).
Jeon, M. Y. et al. Maritoclax enhances TRAIL-induced apoptosis via CHOP-mediated upregulation of DR5 and miR-708-mediated downregulation of cFLIP. Molecules 23(11), 3030. https://doi.org/10.3390/molecules23113030 (2018).
Gu, S., Chen, C., Jiang, X. & Zhang, Z. ROS-mediated endoplasmic reticulum stress and mitochondrial dysfunction underlie apoptosis induced by resveratrol and arsenic trioxide in A549 cells. Chem. Biol. Interact. 245, 100–109. https://doi.org/10.1016/j.cbi.2016.01.005 (2016).
Ma, X., Yan, L., Zhu, Q. & Shao, F. Puerarin attenuates cisplatin-induced rat nephrotoxicity: The involvement of TLR4/NF-κB signaling pathway. PLoS One 12(2), e0171612. https://doi.org/10.1371/journal.pone.0171612 (2017).
Li, W., Yang, Y., Li, Y., Zhao, Y. & Jiang, H. Sirt5 attenuates cisplatin-induced acute kidney injury through regulation of Nrf2/HO-1 and Bcl-2. Biomed. Res. Int. 2019, 4745132. https://doi.org/10.1155/2019/4745132 (2019).
Ogueji, E., Nwani, C., Mbah, C., Iheanacho, S. & Nweke, F. Oxidative stress, biochemical, lipid peroxidation, and antioxidant responses in Clarias gariepinus exposed to acute concentrations of ivermectin. Environ. Sci. Pollut. Res. Int. 27(14), 16806–16815. https://doi.org/10.1007/s11356-019-07035-4 (2020).
Agarwal, A. & Nick, H. S. Renal response to tissue injury: Lessons from heme oxygenase-1 GeneAblation and expression. J. Am. Soc. Nephrol. 11(5), 965–973. https://doi.org/10.1681/ASN.V115965 (2000).
Agarwal, A., Balla, J., Alam, J., Croatt, A. J. & Nath, K. A. Induction of heme oxygenase in toxic renal injury: A protective role in cisplatin nephrotoxicity in the rat. Kidney Int. 48(4), 1298–1307. https://doi.org/10.1038/ki.1995.414 (1995).
Shiraishi, F. et al. Heme oxygenase-1 gene ablation or expression modulates cisplatin-induced renal tubular apoptosis. Am. J. Physiol. Renal Physiol. 278(5), F726–F736. https://doi.org/10.1152/ajprenal.2000.278.5.F726 (2000).
Bolisetty, S. et al. Heme oxygenase-1 inhibits renal tubular macroautophagy in acute kidney injury. J. Am. Soc. Nephrol. 21(10), 1702–1712. https://doi.org/10.1681/ASN.2010030238 (2010).
Nakagawa, T. et al. Caspase-12 mediates endoplasmic-reticulum-specific apoptosis and cytotoxicity by amyloid-beta. Nature 403(6765), 98–103. https://doi.org/10.1038/47513 (2000).
Dille, J. R. Historical development of civil aviation medical standards. Aviat. Space Environ. Med. 63(3), 237–238 (1992).
Zhang, B., Ramesh, G., Uematsu, S. & Akira, S. TLR4 signaling mediates inflammation and tissue injury in nephrotoxicity. J. Am. Soc. Nephrol. 19(5), 923–932. https://doi.org/10.1681/ASN.2007090982 (2008).
Kuhad, A. et al. Effect of curcumin on inflammation and oxidative stress in cisplatin-induced experimental nephrotoxicity. J. Agric. Food Chem. 55(25), 10150–10155. https://doi.org/10.1021/jf0723965 (2007).
Hayase, N. et al. Recombinant thrombomodulin on neutrophil extracellular traps in murine intestinal ischemia–reperfusion. Anesthesiology 131(4), 866–882. https://doi.org/10.1097/ALN.0000000000002898 (2019).
Terryn, S. et al. A primary culture of mouse proximal tubular cells, established on collagen-coated membranes. Am. J. Physiol. Renal Physiol. 293(2), F476–F485. https://doi.org/10.1152/ajprenal.00363.2006 (2007).
Tanaka, K. et al. Pharmacological differentiation of thrombomodulin alfa and activated protein C on coagulation and fibrinolysis in vitro. Clin. Appl. Thromb. Hemost. 24(6), 859–866. https://doi.org/10.1177/1076029618770274 (2018).
Kimura, A. et al. Exaggerated arsenic nephrotoxicity in female mice through estrogen-dependent impairments in the autophagic flux. Toxicology 2(339), 9–18. https://doi.org/10.1016/j.tox.2015.11.005 (2016).
Ishida, Y. et al. Essential involvement of neutrophil elastase in acute acetaminophen hepatotoxicity using BALB/c mice. Int. J. Mol. Sci. 24(9), 7845. https://doi.org/10.3390/ijms24097845 (2023).
- The Renal Warrior Project. Join Now
- Source: https://www.nature.com/articles/s41598-024-64619-y