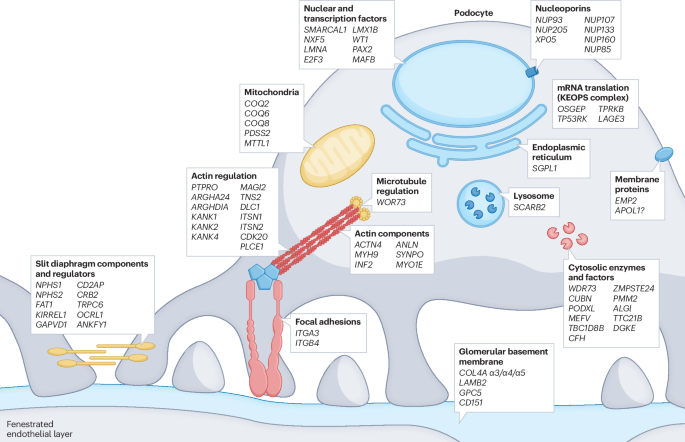
Pavenstadt, H., Kriz, W. & Kretzler, M. Cell biology of the glomerular podocyte. Physiol. Rev. 83, 253–307 (2003).
Abrahamson, D. R. Role of the podocyte (and glomerular endothelium) in building the GBM. Semin. Nephrol. 32, 342–349 (2012).
Srivastava, T. et al. Mechanotransduction signaling in podocytes from fluid flow shear stress. Am. J. Physiol. Ren. Physiol. 314, F22–F34 (2018).
Smoyer, W. E. & Mundel, P. Regulation of podocyte structure during the development of nephrotic syndrome. J. Mol. Med. 76, 172–183 (1998).
Ichimura, K., Kurihara, H. & Sakai, T. Actin filament organization of foot processes in rat podocytes. J. Histochem. Cytochem. 51, 1589–1600 (2003).
Schell, C. & Huber, T. B. The evolving complexity of the podocyte cytoskeleton. J. Am. Soc. Nephrol. 28, 3166–3174 (2017).
Wharram, B. L. et al. Podocyte depletion causes glomerulosclerosis: diphtheria toxin-induced podocyte depletion in rats expressing human diphtheria toxin receptor transgene. J. Am. Soc. Nephrol. 16, 2941–2952 (2005).
Dimke, H., Maezawa, Y. & Quaggin, S. E. Crosstalk in glomerular injury and repair. Curr. Opin. Nephrol. Hypertens. 24, 231–238 (2015).
Xing, C. Y. et al. Direct effects of dexamethasone on human podocytes. Kidney Int. 70, 1038–1045 (2006).
Fornoni, A. et al. Rituximab targets podocytes in recurrent focal segmental glomerulosclerosis. Sci. Transl. Med. 3, 85ra46 (2011).
Faul, C. et al. The actin cytoskeleton of kidney podocytes is a direct target of the antiproteinuric effect of cyclosporine A. Nat. Med. 14, 931–938 (2008).
Dahl, N. K. et al. The clinical utility of genetic testing in the diagnosis and management of adults with chronic kidney disease. J. Am. Soc. Nephrol. 34, 2039–2050 (2023).
Thompson, A. et al. Proteinuria reduction as a surrogate end point in trials of IgA nephropathy. Clin. J. Am. Soc. Nephrol. 14, 469–481 (2019).
Yao, T. et al. Integration of genetic testing and pathology for the diagnosis of adults with FSGS. Clin. J. Am. Soc. Nephrol. 14, 213–223 (2019).
Sampson, M. G. et al. Using population genetics to interrogate the monogenic nephrotic syndrome diagnosis in a case cohort. J. Am. Soc. Nephrol. 27, 1970–1983 (2016).
Trautmann, A., Lipska-Zietkiewicz, B. S. & Schaefer, F. Exploring the clinical and genetic spectrum of steroid resistant nephrotic syndrome: the PodoNet registry. Front. Pediatr. 6, 200 (2018).
Bierzynska, A. et al. Genomic and clinical profiling of a national nephrotic syndrome cohort advocates a precision medicine approach to disease management. Kidney Int. 91, 937–947 (2017).
Buscher, A. K. et al. Immunosuppression and renal outcome in congenital and pediatric steroid-resistant nephrotic syndrome. Clin. J. Am. Soc. Nephrol. 5, 2075–2084 (2010).
Palmer, N. D. & Freedman, B. I. APOL1 and progression of nondiabetic nephropathy. J. Am. Soc. Nephrol. 24, 1344–1346 (2013).
Savin, V. J. et al. Circulating factor associated with increased glomerular permeability to albumin in recurrent focal segmental glomerulosclerosis. N. Engl. J. Med. 334, 878–883 (1996).
Gallon, L., Leventhal, J., Skaro, A., Kanwar, Y. & Alvarado, A. Resolution of recurrent focal segmental glomerulosclerosis after retransplantation. N. Engl. J. Med. 366, 1648–1649 (2012).
Cara-Fuentes, G. et al. CD80 and suPAR in patients with minimal change disease and focal segmental glomerulosclerosis: diagnostic and pathogenic significance. Pediatr. Nephrol. 29, 1363–1371 (2013).
Wei, C. et al. Circulating urokinase receptor as a cause of focal segmental glomerulosclerosis. Nat. Med. 17, 952–960 (2011).
Clement, L. C. et al. Podocyte-secreted angiopoietin-like-4 mediates proteinuria in glucocorticoid-sensitive nephrotic syndrome. Nat. Med. 17, 117–122 (2011).
Sharma, M. et al. Janus kinase 2/signal transducer and activator of transcription 3 inhibitors attenuate the effect of cardiotrophin-like cytokine factor 1 and human focal segmental glomerulosclerosis serum on glomerular filtration barrier. Transl. Res. 166, 384–398 (2015).
Delville, M. et al. A circulating antibody panel for pretransplant prediction of FSGS recurrence after kidney transplantation. Sci. Transl. Med. 6, 256ra136 (2014).
Deegens, J. K. & Wetzels, J. F. Glomerular disease: the search goes on: suPAR is not the elusive FSGS factor. Nat. Rev. Nephrol. 10, 431–432 (2014).
Kronbichler, A., Saleem, M. A., Meijers, B. & Shin, J. I. Soluble urokinase receptors in focal segmental glomerulosclerosis: a review on the scientific point of view. J. Immunol. Res. 2016, 2068691 (2016).
Watts, A. J. B. et al. Discovery of autoantibodies targeting nephrin in minimal change disease supports a novel autoimmune etiology. J. Am. Soc. Nephrol. 33, 238–252 (2022).
Shirai, Y. et al. A multi-institutional study found a possible role of anti-nephrin antibodies in post-transplant focal segmental glomerulosclerosis recurrence. Kidney Int. 105, 608–617 (2023).
Zang, N. et al. cGAS-STING activation contributes to podocyte injury in diabetic kidney disease. iScience 25, 105145 (2022).
Wu, J. et al. The key role of NLRP3 and STING in APOL1-associated podocytopathy. J. Clin. Invest. 131, e136329 (2021).
Yu, B. C. et al. Minimal change disease is associated with mitochondrial injury and STING pathway activation. J. Clin. Med. 11, 577 (2022).
Pedigo, C. E. et al. Local TNF causes NFATc1-dependent cholesterol-mediated podocyte injury. J. Clin. Invest. 126, 3336–3350 (2016).
Idasiak-Piechocka, I., Oko, A., Pawliczak, E., Kaczmarek, E. & Czekalski, S. Urinary excretion of soluble tumour necrosis factor receptor 1 as a marker of increased risk of progressive kidney function deterioration in patients with primary chronic glomerulonephritis. Nephrol. Dial. Transpl. 25, 3948–3956 (2010).
Chung, C. F. et al. Intrinsic tumor necrosis factor-α pathway is activated in a subset of patients with focal segmental glomerulosclerosis. PLoS One 14, e0216426 (2019).
Joy, M. S. et al. Phase 1 trial of adalimumab in focal segmental glomerulosclerosis (FSGS): II. Report of the FONT (Novel Therapies for Resistant FSGS) study group. Am. J. Kidney Dis. 55, 50–60 (2010).
Trachtman, H. et al. Efficacy of galactose and adalimumab in patients with resistant focal segmental glomerulosclerosis: report of the font clinical trial group. BMC Nephrol. 16, 111 (2015).
Zhai, S., Zhao, L., Zhang, Y. & Ma, Q. Interleukin-7 stimulation inhibits nephrin activation and induces podocyte injury. Biochem. Biophys. Res. Commun. 507, 100–105 (2018).
Xiong, T. et al. Interleukin-9 protects from early podocyte injury and progressive glomerulosclerosis in Adriamycin-induced nephropathy. Kidney Int. 98, 615–629 (2020).
Lee, E. Y. et al. The monocyte chemoattractant protein-1/CCR2 loop, inducible by TGF-β, increases podocyte motility and albumin permeability. Am. J. Physiol. Ren. Physiol. 297, F85–F94 (2009).
Mariani, L. H. et al. Precision nephrology identified tumor necrosis factor activation variability in minimal change disease and focal segmental glomerulosclerosis. Kidney Int. 103, 565–579 (2023).
Kim, A. H. et al. B cell-derived IL-4 acts on podocytes to induce proteinuria and foot process effacement. JCI Insight 2, e81836 (2017).
Zhao, H. et al. Role of mitochondrial dysfunction in renal fibrosis promoted by hypochlorite-modified albumin in a remnant kidney model and protective effects of antioxidant peptide SS-31. Eur. J. Pharmacol. 804, 57–67 (2017).
Agrawal, S., Brier, M. E., Kerlin, B. A., Smoyer, W. E. & Pediatric Nephrology Research Consortium. Plasma cytokine profiling to predict steroid resistance in pediatric nephrotic syndrome. Kidney Int. Rep. 6, 785–795 (2021).
Torban, E. et al. From podocyte biology to novel cures for glomerular disease. Kidney Int. 96, 850–861 (2019).
Angeletti, A. et al. Loss of decay-accelerating factor triggers podocyte injury and glomerulosclerosis. J. Exp. Med. 217, e20191699 (2020).
Huang, J. et al. Complement activation profile of patients with primary focal segmental glomerulosclerosis. PLoS One 15, e0234934 (2020).
Thurman, J. M. et al. Complement activation in patients with focal segmental glomerulosclerosis. PLoS One 10, e0136558 (2015).
Lu, Q. et al. Complement factor B in high glucose-induced podocyte injury and diabetic kidney disease. JCI Insight 6, e147716 (2021).
Tonneijck, L. et al. Glomerular hyperfiltration in diabetes: mechanisms, clinical significance, and treatment. J. Am. Soc. Nephrol. 28, 1023–1039 (2017).
Rossitto, G. et al. High sodium intake, glomerular hyperfiltration, and protein catabolism in patients with essential hypertension. Cardiovasc. Res. 117, 1372–1381 (2021).
Srivastava, T. et al. Role of biomechanical forces in hyperfiltration-mediated glomerular injury in congenital anomalies of the kidney and urinary tract. Nephrol. Dial. Transpl. 32, 759–765 (2017).
Holscher, D. L. et al. Next-generation morphometry for pathomics-data mining in histopathology. Nat. Commun. 14, 470 (2023).
Kriz, W. & Lemley, K. V. A potential role for mechanical forces in the detachment of podocytes and the progression of CKD. J. Am. Soc. Nephrol. 26, 258–269 (2015).
Sharma, M., Sharma, R., McCarthy, E. T., Savin, V. J. & Srivastava, T. Hyperfiltration-associated biomechanical forces in glomerular injury and response: potential role for eicosanoids. Prostaglandins Other Lipid Mediat. 132, 59–68 (2017).
Gyarmati, G. et al. The role of TRPC6 calcium channels and P2 purinergic receptors in podocyte mechanical and metabolic sensing. Physiol. Int. https://doi.org/10.1556/2060.2021.00205 (2021).
Dupont, S. et al. Role of YAP/TAZ in mechanotransduction. Nature 474, 179–183 (2011).
Endlich, K., Kliewe, F. & Endlich, N. Stressed podocytes-mechanical forces, sensors, signaling and response. Pflug. Arch. 469, 937–949 (2017).
Sharma, M. et al. Glomerular biomechanical stress and lipid mediators during cellular changes leading to chronic kidney disease. Biomedicines 10, 407 (2022).
Srivastava, T. et al. Prostaglandin E(2) is crucial in the response of podocytes to fluid flow shear stress. J. Cell Commun. Signal. 4, 79–90 (2010).
Liu, S. et al. Single-cell transcriptomics reveals a mechanosensitive injury signaling pathway in early diabetic nephropathy. Genome Med. 15, 2 (2023).
Vallon, V. & Thomson, S. C. The tubular hypothesis of nephron filtration and diabetic kidney disease. Nat. Rev. Nephrol. 16, 317–336 (2020).
Heerspink, H. J., Perkins, B. A., Fitchett, D. H., Husain, M. & Cherney, D. Z. Sodium glucose cotransporter 2 inhibitors in the treatment of diabetes mellitus: cardiovascular and kidney effects, potential mechanisms, and clinical applications. Circulation 134, 752–772 (2016).
Sawada, K. et al. Upregulation of α3β1-integrin in podocytes in early-stage diabetic nephropathy. J. Diabetes Res. 2016, 9265074 (2016).
Iglesias-de la Cruz, M. C. et al. Effects of high glucose and TGF-β1 on the expression of collagen IV and vascular endothelial growth factor in mouse podocytes. Kidney Int. 62, 901–913 (2002).
Rabbani, N. & Thornalley, P. J. Advanced glycation end products in the pathogenesis of chronic kidney disease. Kidney Int. 93, 803–813 (2018).
Lay, A. C. & Coward, R. J. M. The evolving importance of insulin signaling in podocyte health and disease. Front. Endocrinol. 9, 693 (2018).
Eid, A. A. et al. Mechanisms of podocyte injury in diabetes: role of cytochrome P450 and NADPH oxidases. Diabetes 58, 1201–1211 (2009).
Galvan, D. L. et al. Real-time in vivo mitochondrial redox assessment confirms enhanced mitochondrial reactive oxygen species in diabetic nephropathy. Kidney Int. 92, 1282–1287 (2017).
Jha, J. C. et al. Podocyte-specific Nox4 deletion affords renoprotection in a mouse model of diabetic nephropathy. Diabetologia 59, 379–389 (2016).
Jha, J. C. et al. Independent of renox, NOX5 promotes renal inflammation and fibrosis in diabetes by activating ROS-sensitive pathways. Diabetes 71, 1282–1298 (2022).
Chen, A. et al. Soluble RARRES1 induces podocyte apoptosis to promote glomerular disease progression. J. Clin. Invest. 130, 5523–5535 (2020).
Ducasa, G. M. et al. ATP-binding cassette A1 deficiency causes cardiolipin-driven mitochondrial dysfunction in podocytes. J. Clin. Invest. 129, 3387–3400 (2019).
Wright, M. B. et al. Compounds targeting OSBPL7 increase ABCA1-dependent cholesterol efflux preserving kidney function in two models of kidney disease. Nat. Commun. 12, 4662 (2021).
Li, Y. et al. Therapeutic potential targeting podocyte mitochondrial dysfunction in focal segmental glomerulosclerosis. Kidney Dis. 9, 254–264 (2023).
Kaneko, S. et al. Mitochondrial DNA deletion-dependent podocyte injuries in Mito-miceΔ, a murine model of mitochondrial disease. Exp. Anim. 71, 14–21 (2022).
Jiang, L. et al. Calmodulin-dependent protein kinase II/cAMP response element-binding protein/Wnt/β-catenin signaling cascade regulates angiotensin II-induced podocyte injury and albuminuria. J. Biol. Chem. 288, 23368–23379 (2013).
Tian, D. et al. Antagonistic regulation of actin dynamics and cell motility by TRPC5 and TRPC6 channels. Sci. Signal. 3, ra77 (2010).
Struk, T. et al. Transcriptome analysis of primary podocytes reveals novel calcium regulated regulatory networks. FASEB J. 34, 14490–14506 (2020).
Xu, H. et al. IP3R-Grp75-VDAC1-MCU calcium regulation axis antagonists protect podocytes from apoptosis and decrease proteinuria in an Adriamycin nephropathy rat model. BMC Nephrol. 19, 140 (2018).
Yang, Q. et al. Loss of UCP2 causes mitochondrial fragmentation by OMA1-dependent proteolytic processing of OPA1 in podocytes. FASEB J. 37, e23265 (2023).
Yang, Y. Y. et al. Identification of ULK1 as a novel mitophagy-related gene in diabetic nephropathy. Front. Endocrinol. 13, 1079465 (2022).
Kawakami, T. et al. Deficient autophagy results in mitochondrial dysfunction and FSGS. J. Am. Soc. Nephrol. 26, 1040–1052 (2015).
Yildirim, D. et al. Role of autophagy and evaluation the effects of microRNAs 214, 132, 34c and prorenin receptor in a rat model of focal segmental glomerulosclerosis. Life Sci. 280, 119671 (2021).
Liu, B. et al. Zhen-Wu-Tang induced mitophagy to protect mitochondrial function in chronic glomerulonephritis via PI3K/AKT/mTOR and AMPK pathways. Front. Pharmacol. 12, 777670 (2021).
Godel, M. et al. Role of mTOR in podocyte function and diabetic nephropathy in humans and mice. J. Clin. Invest. 121, 2197–2209 (2011).
Cina, D. P. et al. Inhibition of MTOR disrupts autophagic flux in podocytes. J. Am. Soc. Nephrol. 23, 412–420 (2012).
Inoki, K. et al. mTORC1 activation in podocytes is a critical step in the development of diabetic nephropathy in mice. J. Clin. Invest. 121, 2181–2196 (2011).
Ito, N. et al. mTORC1 activation triggers the unfolded protein response in podocytes and leads to nephrotic syndrome. Lab. Invest. 91, 1584–1595 (2011).
Mao, J. et al. Mammalian target of rapamycin complex 1 activation in podocytes promotes cellular crescent formation. Am. J. Physiol. Ren. Physiol. 307, F1023–F1032 (2014).
Munivenkatappa, R. et al. Tubular epithelial cell and podocyte apoptosis with de novo sirolimus based immunosuppression in renal allograft recipients with DGF. Histol. Histopathol. 25, 189–196 (2010).
Cho, M. E., Hurley, J. K. & Kopp, J. B. Sirolimus therapy of focal segmental glomerulosclerosis is associated with nephrotoxicity. Am. J. Kidney Dis. 49, 310–317 (2007).
Tumlin, J. A. et al. A prospective, open-label trial of sirolimus in the treatment of focal segmental glomerulosclerosis. Clin. J. Am. Soc. Nephrol. 1, 109–116 (2006).
Liern, M., De Reyes, V., Fayad, A. & Vallejo, G. Use of sirolimus in patients with primary steroid-resistant nephrotic syndrome. Nefrologia 32, 321–328 (2012).
Kato, H. & Susztak, K. Repair problems in podocytes: Wnt, Notch, and glomerulosclerosis. Semin. Nephrol. 32, 350–356 (2012).
Teixeira Vde, P. et al. Functional consequences of integrin-linked kinase activation in podocyte damage. Kidney Int. 67, 514–523 (2005).
Dai, C. et al. Wnt/β-catenin signaling promotes podocyte dysfunction and albuminuria. J. Am. Soc. Nephrol. 20, 1997–2008 (2009).
Kato, H. et al. Wnt/β-catenin pathway in podocytes integrates cell adhesion, differentiation, and survival. J. Biol. Chem. 286, 26003–26015 (2011).
Lin, C. L. et al. Dickkopf-1 promotes hyperglycemia-induced accumulation of mesangial matrix and renal dysfunction. J. Am. Soc. Nephrol. 21, 124–135 (2010).
Niranjan, T. et al. The Notch pathway in podocytes plays a role in the development of glomerular disease. Nat. Med. 14, 290–298 (2008).
Waters, A. M. et al. Ectopic notch activation in developing podocytes causes glomerulosclerosis. J. Am. Soc. Nephrol. 19, 1139–1157 (2008).
Lai, A. S. & Lai, K. N. Viral nephropathy. Nat. Clin. Pract. Nephrol. 2, 254–262 (2006).
Angeletti, A., Cantarelli, C. & Cravedi, P. HCV-associated nephropathies in the era of direct acting antiviral agents. Front. Med. 6, 20 (2019).
Chan, T. M. Hepatitis B and renal disease. Curr. Hepat. Rep. 9, 99–105 (2010).
Bruggeman, L. A. et al. Renal epithelium is a previously unrecognized site of HIV-1 infection. J. Am. Soc. Nephrol. 11, 2079–2087 (2000).
Canaud, G. et al. The kidney as a reservoir for HIV-1 after renal transplantation. J. Am. Soc. Nephrol. 25, 407–419 (2014).
Winston, J. A. et al. Nephropathy and establishment of a renal reservoir of HIV type 1 during primary infection. N. Engl. J. Med. 344, 1979–1984 (2001).
Genovese, G. et al. Association of trypanolytic ApoL1 variants with kidney disease in African Americans. Science 329, 841–845 (2010).
Friedman, D. J. & Pollak, M. R. APOL1 nephropathy: from genetics to clinical applications. Clin. J. Am. Soc. Nephrol. 16, 294–303 (2021).
Kudose, S. et al. Kidney biopsy findings in patients with COVID-19. J. Am. Soc. Nephrol. 31, 1959–1968 (2020).
Shetty, A. A. et al. COVID-19-associated glomerular disease. J. Am. Soc. Nephrol. 32, 33–40 (2021).
Wu, H. et al. AKI and collapsing glomerulopathy associated with COVID-19 and APOL 1 high-risk genotype. J. Am. Soc. Nephrol. 31, 1688–1695 (2020).
Puelles, V. G. et al. Multiorgan and renal tropism of SARS-CoV-2. N. Engl. J. Med. 383, 590–592 (2020).
Roufosse, C. et al. Electron microscopic investigations in COVID-19: not all crowns are coronas. Kidney Int. 98, 505–506 (2020).
Hassler, L., Reyes, F., Sparks, M. A., Welling, P. & Batlle, D. Evidence for and against direct kidney infection by SARS-CoV-2 in patients with COVID-19. Clin. J. Am. Soc. Nephrol. 16, 1755–1765 (2021).
Pagtalunan, M. E. et al. Podocyte loss and progressive glomerular injury in type II diabetes. J. Clin. Invest. 99, 342–348 (1997).
Lemley, K. V. et al. Podocytopenia and disease severity in IgA nephropathy. Kidney Int. 61, 1475–1485 (2002).
Yin, L., Yu, L., He, J. C. & Chen, A. Controversies in podocyte loss: death or detachment? Front. Cell Dev. Biol. 9, 771931 (2021).
Shankland, S. J., Anders, H. J. & Romagnani, P. Glomerular parietal epithelial cells in kidney physiology, pathology, and repair. Curr. Opin. Nephrol. Hypertens. 22, 302–309 (2013).
Appel, D. et al. Recruitment of podocytes from glomerular parietal epithelial cells. J. Am. Soc. Nephrol. 20, 333–343 (2009).
Lazzeri, E. et al. Regenerative potential of embryonic renal multipotent progenitors in acute renal failure. J. Am. Soc. Nephrol. 18, 3128–3138 (2007).
Sagrinati, C. et al. Isolation and characterization of multipotent progenitor cells from the Bowman’s capsule of adult human kidneys. J. Am. Soc. Nephrol. 17, 2443–2456 (2006).
Ronconi, E. et al. The role of podocyte damage in the pathogenesis of glomerulosclerosis and possible repair mechanisms [Italian]. G. Ital. Nefrol. 26, 660–669 (2009).
Liu, W. B. et al. Single cell landscape of parietal epithelial cells in healthy and diseased states. Kidney Int. 104, 108–123 (2023).
Kaverina, N. V. et al. Tracking the stochastic fate of cells of the renin lineage after podocyte depletion using multicolor reporters and intravital imaging. PLoS One 12, e0173891 (2017).
Lichtnekert, J. et al. Renin-angiotensin-aldosterone system inhibition increases podocyte derivation from cells of renin lineage. J. Am. Soc. Nephrol. 27, 3611–3627 (2016).
Pippin, J. W. et al. Cells of renin lineage are progenitors of podocytes and parietal epithelial cells in experimental glomerular disease. Am. J. Pathol. 183, 542–557 (2013).
Macconi, D. et al. Podocyte repopulation contributes to regression of glomerular injury induced by ACE inhibition. Am. J. Pathol. 174, 797–807 (2009).
Watanabe, H. et al. Inhibition of the renin-angiotensin system causes concentric hypertrophy of renal arterioles in mice and humans. JCI Insight 6, e154337 (2021).
Wanner, N. et al. Unraveling the role of podocyte turnover in glomerular aging and injury. J. Am. Soc. Nephrol. 25, 707–716 (2014).
Dai, Y. et al. Retinoic acid improves nephrotoxic serum-induced glomerulonephritis through activation of podocyte retinoic acid receptor α. Kidney Int. 92, 1444–1457 (2017).
Peired, A. et al. Proteinuria impairs podocyte regeneration by sequestering retinoic acid. J. Am. Soc. Nephrol. 24, 1756–1768 (2013).
Grahammer, F., Wanner, N. & Huber, T. B. Podocyte regeneration: who can become a podocyte? Am. J. Pathol. 183, 333–335 (2013).
Arendshorst, W. J., Brannstrom, K. & Ruan, X. Actions of angiotensin II on the renal microvasculature. J. Am. Soc. Nephrol. 10, S149–S161 (1999).
Casare, F. A. et al. Renovascular remodeling and renal injury after extended angiotensin II infusion. Am. J. Physiol. Ren. Physiol. 310, F1295–F1307 (2016).
Lewis, E. J., Hunsicker, L. G., Bain, R. P. & Rohde, R. D. The effect of angiotensin-converting-enzyme inhibition on diabetic nephropathy. The Collaborative Study Group. N. Engl. J. Med. 329, 1456–1462 (1993).
Lewis, E. J. et al. Renoprotective effect of the angiotensin-receptor antagonist irbesartan in patients with nephropathy due to type 2 diabetes. N. Engl. J. Med. 345, 851–860 (2001).
Gansevoort, R. T., Sluiter, W. J., Hemmelder, M. H., de Zeeuw, D. & de Jong, P. E. Antiproteinuric effect of blood-pressure-lowering agents: a meta-analysis of comparative trials. Nephrol. Dial. Transpl. 10, 1963–1974 (1995).
Apperloo, A. J., de Zeeuw, D., Sluiter, H. E. & de Jong, P. E. Differential effects of enalapril and atenolol on proteinuria and renal haemodynamics in non-diabetic renal disease. BMJ 303, 821–824 (1991).
Ren, Z. et al. Angiotensin II induces nephrin dephosphorylation and podocyte injury: role of caveolin-1. Cell Signal. 24, 443–450 (2012).
Wang, S. et al. Angiotensin II induces reorganization of the actin cytoskeleton and myosin light-chain phosphorylation in podocytes through rho/ROCK-signaling pathway. Ren. Fail. 38, 268–275 (2016).
Cardoso, V. G. et al. Angiotensin II-induced podocyte apoptosis is mediated by endoplasmic reticulum stress/PKC-δ/p38 MAPK pathway activation and trough increased Na+/H+ exchanger isoform 1 activity. BMC Nephrol. 19, 179 (2018).
Tao, Y. et al. Enhanced Orai1-mediated store-operated Ca2+ channel/calpain signaling contributes to high glucose-induced podocyte injury. J. Biol. Chem. 298, 101990 (2022).
Tu, P., Gibon, J. & Bouron, A. The TRPC6 channel activator hyperforin induces the release of zinc and calcium from mitochondria. J. Neurochem. 112, 204–213 (2010).
Binz-Lotter, J. et al. Injured podocytes are sensitized to angiotensin II-induced calcium signaling. J. Am. Soc. Nephrol. 31, 532–542 (2020).
Kidney Disease: Improving Global Outcomes Glomerular Diseases Work Group. KDIGO 2021 clinical practice guideline for the management of glomerular diseases. Kidney Int. 100, S1–S276 (2021).
Heerspink, H. J., Johnsson, E., Gause-Nilsson, I., Cain, V. A. & Sjostrom, C. D. Dapagliflozin reduces albuminuria in patients with diabetes and hypertension receiving renin-angiotensin blockers. Diabetes Obes. Metab. 18, 590–597 (2016).
Cherney, D. et al. The effect of sodium glucose cotransporter 2 inhibition with empagliflozin on microalbuminuria and macroalbuminuria in patients with type 2 diabetes. Diabetologia 59, 1860–1870 (2016).
Barnett, A. H. et al. Efficacy and safety of empagliflozin added to existing antidiabetes treatment in patients with type 2 diabetes and chronic kidney disease: a randomised, double-blind, placebo-controlled trial. Lancet Diabetes Endocrinol. 2, 369–384 (2014).
Yale, J. F. et al. Initiation of once daily insulin detemir is not associated with weight gain in patients with type 2 diabetes mellitus: results from an observational study. Diabetol. Metab. Syndr. 5, 56 (2013).
The EMPA-KIDNEY Collaborative Group et al. Empagliflozin in patients with chronic kidney disease. N. Engl. J. Med. 388, 117–127 (2023).
Wheeler, D. C. et al. Effects of dapagliflozin on major adverse kidney and cardiovascular events in patients with diabetic and non-diabetic chronic kidney disease: a prespecified analysis from the DAPA-CKD trial. Lancet Diabetes Endocrinol. 9, 22–31 (2021).
Wheeler, D. C. et al. A pre-specified analysis of the DAPA-CKD trial demonstrates the effects of dapagliflozin on major adverse kidney events in patients with IgA nephropathy. Kidney Int. 100, 215–224 (2021).
Wheeler, D. C. et al. Safety and efficacy of dapagliflozin in patients with focal segmental glomerulosclerosis: a prespecified analysis of the dapagliflozin and prevention of adverse outcomes in chronic kidney disease (DAPA-CKD) trial. Nephrol. Dial. Transpl. 37, 1647–1656 (2022).
Nuffield Department of Population Health Renal Studies Group; SGLT2 inhibitor Meta-Analysis Cardio-Renal Trialists’ Consortium. Impact of diabetes on the effects of sodium glucose co-transporter-2 inhibitors on kidney outcomes: collaborative meta-analysis of large placebo-controlled trials. Lancet 400, 1788–1801 (2022).
Cassis, P. et al. SGLT2 inhibitor dapagliflozin limits podocyte damage in proteinuric nondiabetic nephropathy. JCI Insight 3, e98720 (2018).
Ge, M. et al. Empagliflozin reduces podocyte lipotoxicity in experimental Alport syndrome. eLife 12, e83353 (2023).
Wu, H., Kirita, Y., Donnelly, E. L. & Humphreys, B. D. Advantages of single-nucleus over single-cell RNA sequencing of adult kidney: rare cell types and novel cell states revealed in fibrosis. J. Am. Soc. Nephrol. 30, 23–32 (2019).
Tomita, I. et al. SGLT2 inhibition mediates protection from diabetic kidney disease by promoting ketone body-induced mTORC1 inhibition. Cell Metab. 32, 404–419.e6 (2020).
Wang, X. X. et al. SGLT2 protein expression is increased in human diabetic nephropathy: SGLT2 protein inhibition decreases renal lipid accumulation, inflammation, and the development of nephropathy in diabetic mice. J. Biol. Chem. 292, 5335–5348 (2017).
Locatelli, M. et al. Empagliflozin protects glomerular endothelial cell architecture in experimental diabetes through the VEGF-A/caveolin-1/PV-1 signaling pathway. J. Pathol. 256, 468–479 (2022).
Kim, J. J. et al. Discoidin domain receptor 1 activation links extracellular matrix to podocyte lipotoxicity in Alport syndrome. EBioMedicine 63, 103162 (2021).
Jiang, L. et al. RNA sequencing analysis of human podocytes reveals glucocorticoid regulated gene networks targeting non-immune pathways. Sci. Rep. 6, 35671 (2016).
Zhou, H. et al. Loss of the podocyte glucocorticoid receptor exacerbates proteinuria after injury. Sci. Rep. 7, 9833 (2017).
Lewko, B. et al. Dexamethasone-dependent modulation of cyclic GMP synthesis in podocytes. Mol. Cell Biochem. 409, 243–253 (2015).
McCaffrey, J. C. et al. Glucocorticoid therapy regulates podocyte motility by inhibition of Rac1. Sci. Rep. 7, 6725 (2017).
Mallipattu, S. K. et al. Kruppel-like factor 15 mediates glucocorticoid-induced restoration of podocyte differentiation markers. J. Am. Soc. Nephrol. 28, 166–184 (2017).
Mallipattu, S. K. et al. Kruppel-like factor 15 (KLF15) is a key regulator of podocyte differentiation. J. Biol. Chem. 287, 19122–19135 (2012).
Agrawal, S. et al. Pioglitazone enhances the beneficial effects of glucocorticoids in experimental nephrotic syndrome. Sci. Rep. 6, 24392 (2016).
Yu, M., Ren, Q. & Yu, S. Y. Role of nephrin phosphorylation inducted by dexamethasone and angiotensin II in podocytes. Mol. Biol. Rep. 41, 3591–3595 (2014).
Ohashi, T., Uchida, K., Uchida, S., Sasaki, S. & Nitta, K. Dexamethasone increases the phosphorylation of nephrin in cultured podocytes. Clin. Exp. Nephrol. 15, 688–693 (2011).
Wan, X. et al. Loss of epithelial membrane protein 2 aggravates podocyte injury via upregulation of caveolin-1. J. Am. Soc. Nephrol. 27, 1066–1075 (2016).
Zhou, T. et al. Implication of dysregulation of the canonical wingless-type MMTV integration site (WNT) pathway in diabetic nephropathy. Diabetologia 55, 255–266 (2012).
Srivastava, S. P. et al. Loss of endothelial glucocorticoid receptor accelerates diabetic nephropathy. Nat. Commun. 12, 2368 (2021).
Wu, X. et al. Glucocorticoids inhibit EGFR signaling activation in podocytes in anti-GBM crescentic glomerulonephritis. Front. Med. 9, 697443 (2022).
Shi, S. et al. Podocyte-selective deletion of dicer induces proteinuria and glomerulosclerosis. J. Am. Soc. Nephrol. 19, 2159–2169 (2008).
Wu, J. et al. Downregulation of microRNA-30 facilitates podocyte injury and is prevented by glucocorticoids. J. Am. Soc. Nephrol. 25, 92–104 (2014).
Aramburu, J., Heitman, J. & Crabtree, G. R. Calcineurin: a central controller of signalling in eukaryotes. EMBO Rep. 5, 343–348 (2004).
Massella, L. et al. Cyclosporine A treatment in patients with Alport syndrome: a single-center experience. Pediatr. Nephrol. 25, 1269–1275 (2010).
Wu, J. et al. MicroRNA-30 family members regulate calcium/calcineurin signaling in podocytes. J. Clin. Invest. 125, 4091–4106 (2015).
Shen, X. et al. Calcineurin inhibitors cyclosporin A and tacrolimus protect against podocyte injury induced by puromycin aminonucleoside in rodent models. Sci. Rep. 6, 32087 (2016).
Jeruschke, S., Alex, D., Hoyer, P. F. & Weber, S. Protective effects of rituximab on puromycin-induced apoptosis, loss of adhesion and cytoskeletal alterations in human podocytes. Sci. Rep. 12, 12297 (2022).
US National Library of Medicine. ClinicalTrials.gov https://clinicaltrials.gov/ct2/show/NCT04983888 (2021).
Aghajan, M. et al. Antisense oligonucleotide treatment ameliorates IFN-γ-induced proteinuria in APOL1-transgenic mice. JCI Insight 4, e126124 (2019).
Yang, Y. W. et al. Antisense oligonucleotides ameliorate kidney dysfunction in podocyte-specific APOL1 risk variant mice. Mol. Ther. 30, 2491–2504 (2022).
Olabisi, O. A. & Heneghan, J. F. APOL1 nephrotoxicity: what does ion transport have to do with it? Semin. Nephrol. 37, 546–551 (2017).
Olabisi, O. A. et al. APOL1 kidney disease risk variants cause cytotoxicity by depleting cellular potassium and inducing stress-activated protein kinases. Proc. Natl Acad. Sci. USA 113, 830–837 (2016).
Schaub, C. et al. Cation channel conductance and pH gating of the innate immunity factor APOL1 are governed by pore-lining residues within the C-terminal domain. J. Biol. Chem. 295, 13138–13149 (2020).
US National Library of Medicine. ClinicalTrials.gov https://clinicaltrials.gov/ct2/show/NCT05312879 (2022).
He, J. C. et al. Nef stimulates proliferation of glomerular podocytes through activation of Src-dependent Stat3 and MAPK1,2 pathways. J. Clin. Invest. 114, 643–651 (2004).
Meliambro, K. et al. Molecular analysis of the kidney from a patient with COVID-19-associated collapsing glomerulopathy. Kidney Med. 3, 653–658 (2021).
Nystrom, S. E. et al. JAK inhibitor blocks COVID-19 cytokine-induced JAK/STAT/APOL1 signaling in glomerular cells and podocytopathy in human kidney organoids. JCI Insight 7, e157432 (2022).
US National Library of Medicine. ClinicalTrials.gov https://clinicaltrials.gov/ct2/show/NCT05237388 (2023).
Kohan, D. E., Rossi, N. F., Inscho, E. W. & Pollock, D. M. Regulation of blood pressure and salt homeostasis by endothelin. Physiol. Rev. 91, 1–77 (2011).
Morigi, M. et al. In response to protein load podocytes reorganize cytoskeleton and modulate endothelin-1 gene: implication for permselective dysfunction of chronic nephropathies. Am. J. Pathol. 166, 1309–1320 (2005).
Saleh, M. A., Boesen, E. I., Pollock, J. S., Savin, V. J. & Pollock, D. M. Endothelin-1 increases glomerular permeability and inflammation independent of blood pressure in the rat. Hypertension 56, 942–949 (2010).
Lenoir, O. et al. Direct action of endothelin-1 on podocytes promotes diabetic glomerulosclerosis. J. Am. Soc. Nephrol. 25, 1050–1062 (2014).
Saleh, M. A., Pollock, J. S. & Pollock, D. M. Distinct actions of endothelin A-selective versus combined endothelin A/B receptor antagonists in early diabetic kidney disease. J. Pharmacol. Exp. Ther. 338, 263–270 (2011).
Daehn, I. et al. Endothelial mitochondrial oxidative stress determines podocyte depletion in segmental glomerulosclerosis. J. Clin. Invest. 124, 1608–1621 (2014).
Ebefors, K. et al. Endothelin receptor-A mediates degradation of the glomerular endothelial surface layer via pathologic crosstalk between activated podocytes and glomerular endothelial cells. Kidney Int. 96, 957–970 (2019).
Komers, R. & Plotkin, H. Dual inhibition of renin-angiotensin-aldosterone system and endothelin-1 in treatment of chronic kidney disease. Am. J. Physiol. Regul. Integr. Comp. Physiol. 310, R877–R884 (2016).
Trachtman, H. et al. DUET: a phase 2 study evaluating the efficacy and safety of sparsentan in patients with FSGS. J. Am. Soc. Nephrol. 29, 2745–2754 (2018).
Rheault, M. N. et al. Sparsentan versus irbesartan in focal segmental glomerulosclerosis. N. Engl. J. Med. 389, 2436–2445 (2023).
Heerspink, H. J. L. et al. Sparsentan in patients with IgA nephropathy: a prespecified interim analysis from a randomised, double-blind, active-controlled clinical trial. Lancet 401, 1584–1594 (2023).
Syed, Y. Y. Sparsentan: first approval. Drugs 83, 563–568 (2023).
Heerspink, H. J. L. et al. Atrasentan and renal events in patients with type 2 diabetes and chronic kidney disease (SONAR): a double-blind, randomised, placebo-controlled trial. Lancet 393, 1937–1947 (2019).
US National Library of Medicine. ClinicalTrials.gov https://clinicaltrials.gov/ct2/show/NCT05213624 (2022).
Wieder, N. & Greka, A. Calcium, TRPC channels, and regulation of the actin cytoskeleton in podocytes: towards a future of targeted therapies. Pediatr. Nephrol. 31, 1047–1054 (2016).
Krall, P. et al. Podocyte-specific overexpression of wild type or mutant trpc6 in mice is sufficient to cause glomerular disease. PLoS One 5, e12859 (2010).
Winn, M. P. et al. A mutation in the TRPC6 cation channel causes familial focal segmental glomerulosclerosis. Science 308, 1801–1804 (2005).
Moller, C. C. et al. Induction of TRPC6 channel in acquired forms of proteinuric kidney disease. J. Am. Soc. Nephrol. 18, 29–36 (2007).
Shengyou, Y. & Li, Y. The effects of siRNA-silenced TRPC6 on podocyte autophagy and apoptosis induced by AngII. J. Renin Angiotensin Aldosterone Syst. 16, 1266–1273 (2015).
Riehle, M. et al. TRPC6 G757D loss-of-function mutation associates with FSGS. J. Am. Soc. Nephrol. 27, 2771–2783 (2016).
Batool, L. et al. An inactivating human TRPC6 channel mutation without focal segmental glomerulosclerosis. Cell Mol. Life Sci. 80, 265 (2023).
Walsh, L. et al. Safety and efficacy of GFB-887, a TRPC5 channel inhibitor, in patients with focal segmental glomerulosclerosis, treatment-resistant minimal change disease, or diabetic nephropathy: TRACTION-2 trial design. Kidney Int. Rep. 6, 2575–2584 (2021).
Fremeaux-Bacchi, V. et al. Genetics and outcome of atypical hemolytic uremic syndrome: a nationwide French series comparing children and adults. Clin. J. Am. Soc. Nephrol. 8, 554–562 (2013).
Gou, S. J., Yuan, J., Wang, C., Zhao, M. H. & Chen, M. Alternative complement pathway activation products in urine and kidneys of patients with ANCA-associated GN. Clin. J. Am. Soc. Nephrol. 8, 1884–1891 (2013).
Brilland, B. et al. Complement alternative pathway in ANCA-associated vasculitis: two decades from bench to bedside. Autoimmun. Rev. 19, 102424 (2020).
Riedl, M., Thorner, P. & Licht, C. C3 glomerulopathy. Pediatr. Nephrol. 32, 43–57 (2017).
Meuleman, M. S. et al. Rare variants in complement gene in C3 glomerulopathy and immunoglobulin-mediated membranoproliferative GN. Clin. J. Am. Soc. Nephrol. 18, 1435–1445 (2023).
Smith, R. J. H. et al. C3 glomerulopathy — understanding a rare complement-driven renal disease. Nat. Rev. Nephrol. 15, 129–143 (2019).
Rizk, D. V. et al. The emerging role of complement proteins as a target for therapy of IgA nephropathy. Front. Immunol. 10, 504 (2019).
Medjeral-Thomas, N. R., Cook, H. T. & Pickering, M. C. Complement activation in IgA nephropathy. Semin. Immunopathol. 43, 679–690 (2021).
Donadelli, R. et al. Unraveling the molecular mechanisms underlying complement dysregulation by nephritic factors in C3G and IC-MPGN. Front. Immunol. 9, 2329 (2018).
Birmingham, D. J. & Hebert, L. A. The complement system in lupus nephritis. Semin. Nephrol. 35, 444–454 (2015).
Weinstein, A., Alexander, R. V. & Zack, D. J. A review of complement activation in SLE. Curr. Rheumatol. Rep. 23, 16 (2021).
Seifert, L. et al. The classical pathway triggers pathogenic complement activation in membranous nephropathy. Nat. Commun. 14, 473 (2023).
Gao, S., Cui, Z. & Zhao, M. H. Complement C3a and C3a receptor activation mediates podocyte injuries in the mechanism of primary membranous nephropathy. J. Am. Soc. Nephrol. 33, 1742–1756 (2022).
Anwar, I. J. et al. Complement-targeted therapies in kidney transplantation-insights from preclinical studies. Front. Immunol. 13, 984090 (2022).
Filippone, E. J., McCue, P. A. & Farber, J. L. Transplant glomerulopathy. Mod. Pathol. 31, 235–252 (2018).
Bonventre, J. V. Complement and renal ischemia-reperfusion injury. Am. J. Kidney Dis. 38, 430–436 (2001).
Wang, L. et al. Labile heme aggravates renal inflammation and complement activation after ischemia reperfusion injury. Front. Immunol. 10, 2975 (2019).
Legendre, C. M. et al. Terminal complement inhibitor eculizumab in atypical hemolytic-uremic syndrome. N. Engl. J. Med. 368, 2169–2181 (2013).
Bomback, A. S. et al. Eculizumab for dense deposit disease and C3 glomerulonephritis. Clin. J. Am. Soc. Nephrol. 7, 748–756 (2012).
Lee, A. Avacopan: first approval. Drugs 82, 79–85 (2022).
Jayne, D. R. W., Merkel, P. A., Schall, T. J., Bekker, P. & ADVOCATE Study Group. Avacopan for the treatment of ANCA-associated vasculitis. N. Engl. J. Med. 384, 599–609 (2021).
Trachtman, H. Emerging drugs for treatment of focal segmental glomerulosclerosis. Expert Opin. Emerg. Drugs 25, 367–375 (2020).
US National Library of Medicine. ClinicalTrials.gov https://clinicaltrials.gov/ct2/show/NCT05314231 (2022).
Nie, S. et al. Biology drives the discovery of bispecific antibodies as innovative therapeutics. Antib. Ther. 3, 18–62 (2020).
Wooden, B., Tarragon, B., Navarro-Torres, M. & Bomback, A. S. Complement inhibitors for kidney disease. Nephrol. Dial. Transpl. 38, ii29–ii39 (2023).
Hou, Y. et al. Mitochondria-targeted peptide SS-31 attenuates renal injury via an antioxidant effect in diabetic nephropathy. Am. J. Physiol. Ren. Physiol. 310, F547–F559 (2016).
Eirin, A. et al. Mitochondrial protection restores renal function in swine atherosclerotic renovascular disease. Cardiovasc. Res. 103, 461–472 (2014).
US National Library of Medicine. ClinicalTrials.gov https://clinicaltrials.gov/ct2/show/NCT02436447 (2015).
Zhu, Y. et al. Protective effects of inhibition of mitochondrial fission on organ function after sepsis. Front. Pharmacol. 12, 712489 (2021).
Brooks, C., Wei, Q., Cho, S. G. & Dong, Z. Regulation of mitochondrial dynamics in acute kidney injury in cell culture and rodent models. J. Clin. Invest. 119, 1275–1285 (2009).
Cassina, L., Chiaravalli, M. & Boletta, A. Increased mitochondrial fragmentation in polycystic kidney disease acts as a modifier of disease progression. FASEB J. 34, 6493–6507 (2020).
Tanriover, C. et al. The mitochondrion: a promising target for kidney disease. Pharmaceutics 15, 270 (2023).
Lindblom, R. S. J. et al. Delineating a role for the mitochondrial permeability transition pore in diabetic kidney disease by targeting cyclophilin D. Clin. Sci. 134, 239–259 (2020).
Wang, Z. et al. Pharmacological targeting of GSK3β confers protection against podocytopathy and proteinuria by desensitizing mitochondrial permeability transition. Br. J. Pharmacol. 172, 895–909 (2015).
Hong, Q. et al. Increased podocyte Sirtuin-1 function attenuates diabetic kidney injury. Kidney Int. 93, 1330–1343 (2018).
Wang, X. et al. Role of SIRT1 in HIV-associated kidney disease. Am. J. Physiol. Ren. Physiol. 319, F335–F344 (2020).
Sattarinezhad, A., Roozbeh, J., Shirazi Yeganeh, B., Omrani, G. R. & Shams, M. Resveratrol reduces albuminuria in diabetic nephropathy: a randomized double-blind placebo-controlled clinical trial. Diabetes Metab. 45, 53–59 (2019).
Xiao, L. et al. The mitochondria-targeted antioxidant MitoQ ameliorated tubular injury mediated by mitophagy in diabetic kidney disease via Nrf2/PINK1. Redox Biol. 11, 297–311 (2017).
Ahmadi, A. et al. Randomized crossover clinical trial of coenzyme Q10 and nicotinamide riboside in chronic kidney disease. JCI Insight 8, e167274 (2023).
Drovandi, S. et al. Oral Coenzyme Q10 supplementation leads to better preservation of kidney function in steroid-resistant nephrotic syndrome due to primary Coenzyme Q10 deficiency. Kidney Int. 102, 604–612 (2022).
Bagherniya, M. et al. The use of curcumin for the treatment of renal disorders: a systematic review of randomized controlled trials. Adv. Exp. Med. Biol. 1291, 327–343 (2021).
Vanaie, A. et al. Curcumin as a major active component of turmeric attenuates proteinuria in patients with overt diabetic nephropathy. J. Res. Med. Sci. 24, 77 (2019).
Weir, M. A. et al. The effect of micro-particle curcumin on chronic kidney disease progression: the MPAC-CKD randomized clinical trial. Nephrol. Dial. Transpl. 38, 2192–2200 (2023).
Lindell, E., Zhong, L. & Zhang, X. Quiescent cancer cells — a potential therapeutic target to overcome tumor resistance and relapse. Int. J. Mol. Sci. 24, 3762 (2023).
Campbell, K. N. et al. Yes-associated protein (YAP) promotes cell survival by inhibiting proapoptotic dendrin signaling. J. Biol. Chem. 288, 17057–17062 (2013).
Zhong, F. et al. TYRO3 agonist as therapy for glomerular disease. JCI Insight 8, e165207 (2023).
Smart, S. K., Vasileiadi, E., Wang, X., DeRyckere, D. & Graham, D. K. The emerging role of TYRO3 as a therapeutic target in cancer. Cancers 10, 474 (2018).
Lin, B., Ma, Y. Y. & Wang, J. W. Nano-technological approaches for targeting kidney diseases with focus on diabetic nephropathy: recent progress, and future perspectives. Front. Bioeng. Biotechnol. 10, 870049 (2022).
Sabiu, G. et al. Targeted nanotherapy for kidney diseases: a comprehensive review. Nephrol. Dial. Transpl. 38, 1385–1396 (2023).
Wu, L. et al. Albumin-based nanoparticles as methylprednisolone carriers for targeted delivery towards the neonatal Fc receptor in glomerular podocytes. Int. J. Mol. Med. 39, 851–860 (2017).
Bruni, R. et al. Ultrasmall polymeric nanocarriers for drug delivery to podocytes in kidney glomerulus. J. Control. Rel. 255, 94–107 (2017).
Zhan, X. et al. Celastrol antagonizes high glucose-evoked podocyte injury, inflammation and insulin resistance by restoring the HO-1-mediated autophagy pathway. Mol. Immunol. 104, 61–68 (2018).
Wu, M. et al. Celastrol aggravates LPS-induced inflammation and injuries of liver and kidney in mice. Am. J. Transl. Res. 10, 2078–2086 (2018).
Alallam, B., Choukaife, H., Seyam, S., Lim, V. & Alfatama, M. Advanced drug delivery systems for renal disorders. Gels 9, 115 (2023).
Huang, X., Ma, Y., Li, Y., Han, F. & Lin, W. Targeted drug delivery systems for kidney diseases. Front. Bioeng. Biotechnol. 9, 683247 (2021).
Vives, E., Brodin, P. & Lebleu, B. A truncated HIV-1 Tat protein basic domain rapidly translocates through the plasma membrane and accumulates in the cell nucleus. J. Biol. Chem. 272, 16010–16017 (1997).
Arif, E. et al. Slit diaphragm protein Neph1 and its signaling: a novel therapeutic target for protection of podocytes against glomerular injury. J. Biol. Chem. 289, 9502–9518 (2014).
Belmadani, S. et al. A thrombospondin-1 antagonist of transforming growth factor-β activation blocks cardiomyopathy in rats with diabetes and elevated angiotensin II. Am. J. Pathol. 171, 777–789 (2007).
Grange, C. & Bussolati, B. Extracellular vesicles in kidney disease. Nat. Rev. Nephrol. 18, 499–513 (2022).
Mason, W. J. et al. Systemic gene therapy with thymosin β4 alleviates glomerular injury in mice. Sci. Rep. 12, 12172 (2022).
Daga, S. et al. New frontiers to cure Alport syndrome: COL4A3 and COL4A5 gene editing in podocyte-lineage cells. Eur. J. Hum. Genet. 28, 480–490 (2020).
Ding, W. Y. et al. Adeno-associated virus gene therapy prevents progression of kidney disease in genetic models of nephrotic syndrome. Sci. Transl. Med. 15, eabc8226 (2023).
De Vriese, A. S., Wetzels, J. F., Glassock, R. J., Sethi, S. & Fervenza, F. C. Therapeutic trials in adult FSGS: lessons learned and the road forward. Nat. Rev. Nephrol. 17, 619–630 (2021).
Musante, L. et al. Circulating anti-actin and anti-ATP synthase antibodies identify a sub-set of patients with idiopathic nephrotic syndrome. Clin. Exp. Immunol. 141, 491–499 (2005).
Cara-Fuentes, G. et al. Angiopoietin-like-4 and minimal change disease. PLoS One 12, e0176198 (2017).
Jacobs-Cacha, C. et al. A misprocessed form of Apolipoprotein A-I is specifically associated with recurrent focal segmental glomerulosclerosis. Sci. Rep. 10, 1159 (2020).
Lopez-Hellin, J. et al. A form of apolipoprotein a-I is found specifically in relapses of focal segmental glomerulosclerosis following transplantation. Am. J. Transpl. 13, 493–500 (2013).
Alachkar, N., Gupta, G. & Montgomery, R. A. Angiotensin antibodies and focal segmental glomerulosclerosis. N. Engl. J. Med. 368, 971–973 (2013).
Abuzeineh, M., Aala, A., Alasfar, S. & Alachkar, N. Angiotensin II receptor 1 antibodies associate with post-transplant focal segmental glomerulosclerosis and proteinuria. BMC Nephrol. 21, 253 (2020).
Savin, V. J. et al. Renal and hematological effects of CLCF-1, a B-cell-stimulating cytokine of the IL-6 family. J. Immunol. Res. 2015, 714964 (2015).
Wei, C., Sigdel, T. K., Sarwal, M. M. & Reiser, J. Circulating CD40 autoantibody and suPAR synergy drives glomerular injury. Ann. Transl. Med. 3, 300 (2015).
Cara-Fuentes, G., Clapp, W. L., Johnson, R. J. & Garin, E. H. Pathogenesis of proteinuria in idiopathic minimal change disease: molecular mechanisms. Pediatr. Nephrol. 31, 2179–2189 (2016).
Garin, E. H. et al. Urinary CD80 excretion increases in idiopathic minimal-change disease. J. Am. Soc. Nephrol. 20, 260–266 (2009).
Garin, E. H. et al. Urinary CD80 is elevated in minimal change disease but not in focal segmental glomerulosclerosis. Kidney Int. 78, 296–302 (2010).
Yu, C. C. et al. Abatacept in B7-1-positive proteinuric kidney disease. N. Engl. J. Med. 369, 2416–2423 (2013).
Zen, R. C. et al. Urinary CD80 and serum suPAR as biomarkers of glomerular disease among adults in Brazil. Diagnostics 13, 203 (2023).
Lennon, R. et al. Hemopexin induces nephrin-dependent reorganization of the actin cytoskeleton in podocytes. J. Am. Soc. Nephrol. 19, 2140–2149 (2008).
Pukajlo-Marczyk, A. & Zwolinska, D. Involvement of hemopexin in the pathogenesis of proteinuria in children with idiopathic nephrotic syndrome. J. Clin. Med. 10, 3160 (2021).
Holt, R. C. et al. Heparanase activity is dysregulated in children with steroid-sensitive nephrotic syndrome. Kidney Int. 67, 122–129 (2005).
Youssef, D. M., Elbehidy, R. M., El-Shal, A. S. & Sherief, L. M. T helper 1 and T helper 2 cytokines in atopic children with steroid-sensitive nephrotic syndrome. Iran. J. Kidney Dis. 9, 298–305 (2015).
Garin, E. H., Blanchard, D. K., Matsushima, K. & Djeu, J. Y. IL-8 production by peripheral blood mononuclear cells in nephrotic patients. Kidney Int. 45, 1311–1317 (1994).
Garin, E. H., Laflam, P. & Chandler, L. Anti-interleukin 8 antibody abolishes effects of lipoid nephrosis cytokine. Pediatr. Nephrol. 12, 381–385 (1998).
Al-Eisa, A. A., Al Rushood, M. & Al-Attiyah, R. J. Urinary excretion of IL-1β, IL-6 and IL-8 cytokines during relapse and remission of idiopathic nephrotic syndrome. J. Inflamm. Res. 10, 1–5 (2017).
Tain, Y. L., Chen, T. Y. & Yang, K. D. Implications of serum TNF-β and IL-13 in the treatment response of childhood nephrotic syndrome. Cytokine 21, 155–159 (2003).
Yap, H. K. et al. Th1 and Th2 cytokine mRNA profiles in childhood nephrotic syndrome: evidence for increased IL-13 mRNA expression in relapse. J. Am. Soc. Nephrol. 10, 529–537 (1999).
Chebotareva, N. et al. A pilot study of anti-nephrin antibodies in podocytopaties among adults. Nephrology 29, 86–92 (2024).
Charba, D. S. et al. Antibodies to protein tyrosine phosphatase receptor type O (PTPro) increase glomerular albumin permeability (Palb). Am. J. Physiol. Ren. Physiol. 297, F138–F144 (2009).
Beaudreuil, S. et al. Circulating CASK is associated with recurrent focal segmental glomerulosclerosis after transplantation. PLoS One 14, e0219353 (2019).
Zhang, X. et al. CASK, the soluble glomerular permeability factor, is secreted by macrophages in patients with recurrent focal and segmental glomerulo-sclerosis. Front. Immunol. 11, 875 (2020).
Wei, C., Spear, R., Hahm, E. & Reiser, J. suPAR, a circulating kidney disease factor. Front. Med. 8, 745838 (2021).
US National Library of Medicine. ClinicalTrials.gov https://clinicaltrials.gov/ct2/show/NCT05003986 (2021).
Egbuna, O. et al. Inaxaplin for proteinuric kidney disease in persons with two APOL1 variants. N. Engl. J. Med. 388, 969–979 (2023).
US National Library of Medicine. ClinicalTrials.gov https://clinicaltrials.gov/ct2/show/NCT05267262 (2022).
US National Library of Medicine. ClinicalTrials.gov https://clinicaltrials.gov/ct2/show/NCT05183646 (2022).
US National Library of Medicine. ClinicalTrials.gov https://clinicaltrials.gov/ct2/show/NCT05599815 (2023).
US National Library of Medicine. ClinicalTrials.gov https://clinicaltrials.gov/ct2/show/NCT05383547 (2022).
US National Library of Medicine. ClinicalTrials.gov https://clinicaltrials.gov/ct2/show/NCT05441826 (2022).
US National Library of Medicine. ClinicalTrials.gov https://clinicaltrials.gov/ct2/show/NCT04009668 (2019).
US National Library of Medicine. ClinicalTrials.gov https://clinicaltrials.gov/ct2/show/NCT05588063 (2022).
US National Library of Medicine. ClinicalTrials.gov https://clinicaltrials.gov/ct2/show/NCT05583942 (2022).
US National Library of Medicine. ClinicalTrials.gov https://clinicaltrials.gov/ct2/show/NCT04065438 (2020).
US National Library of Medicine. ClinicalTrials.gov https://clinicaltrials.gov/ct2/show/NCT02235857 (2015).
- The Renal Warrior Project. Join Now
- Source: https://www.nature.com/articles/s41581-024-00843-z